7.2 Transcription and Gene Expression Answers
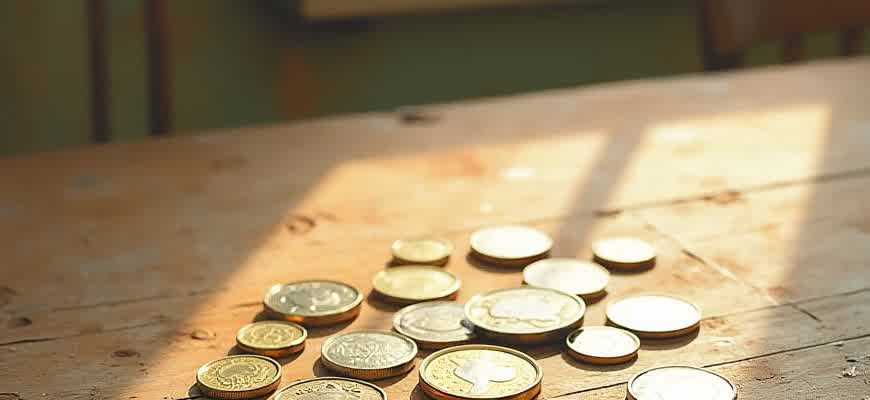
The process of gene expression involves the transcription of DNA into RNA, followed by the translation of that RNA into proteins. Transcription serves as the first step in this pathway, where an RNA molecule is synthesized using a DNA template. This process occurs within the nucleus and is tightly regulated to ensure proper cellular function. Key stages in transcription include initiation, elongation, and termination, each involving distinct molecular machinery.
Understanding transcription requires familiarity with various factors that influence gene activity. Here are some critical elements involved:
- RNA polymerase: The enzyme responsible for synthesizing RNA from a DNA template.
- Promoters: DNA sequences that signal the start of transcription.
- Transcription factors: Proteins that help regulate the initiation of transcription.
"Transcription factors bind to specific DNA sequences, influencing the rate of transcription and thus controlling gene expression."
Following transcription, the RNA produced undergoes various modifications before it can be translated into proteins. These modifications, including splicing and the addition of protective caps and tails, are essential for the stability and functionality of the RNA molecules.
Key stages in gene expression:
- Transcription: RNA is synthesized from the DNA template.
- RNA Processing: Modifications such as capping, polyadenylation, and splicing occur.
- Translation: mRNA is translated into a protein at the ribosome.
Understanding how transcription and gene expression are regulated provides insights into cellular processes and the potential for therapeutic interventions.
Understanding the Role of Transcription in Gene Expression
Transcription is a crucial step in the process of gene expression, where the information encoded in a gene's DNA is copied into messenger RNA (mRNA). This mRNA then serves as a template for protein synthesis, which is essential for cellular functions. Transcription is controlled by various transcription factors and regulatory sequences, ensuring that specific genes are expressed at the right time and in the right cell type.
The process begins when RNA polymerase binds to the promoter region of a gene. This enzyme then synthesizes an mRNA molecule by using one strand of DNA as a template. Several stages of transcription include initiation, elongation, and termination. Each stage is tightly regulated to ensure proper gene expression and to prevent errors that could lead to diseases like cancer.
Key Stages of Transcription
- Initiation: RNA polymerase attaches to the promoter region and unwinds the DNA.
- Elongation: RNA polymerase moves along the DNA strand, synthesizing mRNA.
- Termination: The RNA polymerase reaches a termination sequence and releases the newly formed mRNA.
Factors Influencing Transcription
- Transcription Factors: These are proteins that help RNA polymerase bind to the DNA and begin transcription.
- Enhancers and Silencers: Regulatory elements that increase or decrease the transcription of specific genes.
- Chromatin Structure: The packing of DNA into chromatin can affect the accessibility of the gene to the transcription machinery.
Transcription is not just a simple copying process; it involves complex interactions between DNA, RNA polymerase, and various regulatory factors to ensure accurate gene expression.
Transcription in Eukaryotic Cells
Process | Eukaryotic Cells |
---|---|
Location | Nucleus |
mRNA Processing | Splicing, capping, and polyadenylation occur before export to the cytoplasm |
Post-Transcriptional Regulation | mRNA stability and translation efficiency can be regulated |
How Transcription Factors Influence Gene Activation
Transcription factors are essential regulatory proteins that play a key role in gene expression. They bind to specific regions of DNA, known as promoters or enhancers, to either activate or repress the transcription process. By interacting with the transcriptional machinery, these factors determine which genes are expressed at a given time and to what extent. The precise control of gene expression is critical for cellular function, development, and response to environmental stimuli.
Transcription factors can influence gene activation through various mechanisms, including the recruitment of co-activators or co-repressors, modification of chromatin structure, and direct interaction with the RNA polymerase complex. These factors often work in combination with other signaling pathways, amplifying or dampening the activation process in response to internal and external signals.
Mechanisms of Transcription Factor Action
- Binding to Promoters and Enhancers: Transcription factors bind to specific DNA sequences in promoters or enhancers, influencing the initiation of transcription.
- Chromatin Remodeling: Some transcription factors recruit enzymes that modify chromatin structure, making DNA more or less accessible to the transcriptional machinery.
- Interaction with Co-activators: Transcription factors can recruit co-activators that help assemble the transcription complex or enhance RNA polymerase activity.
Key Elements in Transcription Factor-Mediated Activation
- DNA Binding Domain: The domain responsible for recognizing and binding to specific DNA sequences.
- Activation Domain: A region that interacts with other proteins to activate gene expression.
- Response Elements: Specific DNA sequences within the promoter or enhancer region that are recognized by transcription factors.
Transcription factors are not only important in normal cellular processes but also play critical roles in disease development, including cancer and genetic disorders, where their dysfunction leads to misregulation of gene expression.
Impact on Gene Expression Regulation
Factor | Function | Impact on Gene Expression |
---|---|---|
Activator | Enhances the transcription process | Increases gene expression by facilitating RNA polymerase binding |
Repressor | Blocks transcription initiation | Decreases or silences gene expression by preventing RNA polymerase binding |
Exploring the Mechanisms of mRNA Synthesis
The process of mRNA synthesis is a critical step in gene expression, converting the genetic code stored in DNA into a functional messenger RNA (mRNA) molecule. This mRNA serves as the template for protein synthesis in ribosomes. The transcription process begins when the enzyme RNA polymerase binds to a specific sequence of DNA known as the promoter. This interaction initiates the unwinding of the DNA strands, allowing the RNA polymerase to read the template strand and synthesize a complementary RNA molecule.
Once transcription is initiated, RNA polymerase moves along the DNA template strand, synthesizing an RNA strand in the 5’ to 3’ direction. This synthesis is guided by base-pairing rules where adenine (A) pairs with uracil (U), cytosine (C) pairs with guanine (G), and guanine (G) pairs with cytosine (C). The resulting mRNA is a direct complement of the DNA template strand, except that thymine (T) in DNA is replaced with uracil (U) in RNA.
Key Steps in mRNA Synthesis
- Promoter Recognition: RNA polymerase binds to the promoter region of the gene.
- Transcription Initiation: RNA polymerase unwinds the DNA and begins synthesizing the RNA strand.
- Elongation: RNA polymerase moves along the DNA template, extending the RNA strand.
- Termination: Once the RNA polymerase reaches a termination sequence, the RNA transcript is released.
The process of transcription is essential for converting genetic information into functional proteins. The accuracy of RNA synthesis ensures the proper expression of genes.
Important Enzymes and Factors in Transcription
Enzyme/Factor | Function |
---|---|
RNA Polymerase | Synthesizes the mRNA molecule by reading the DNA template. |
Transcription Factors | Help RNA polymerase bind to the promoter and initiate transcription. |
Activator Proteins | Enhance transcription by binding to specific DNA sequences and promoting RNA polymerase activity. |
- RNA Processing: After transcription, the mRNA undergoes modifications like the addition of a 5’ cap and a poly-A tail.
- Splicing: Introns are removed, and exons are joined together to form the final mRNA sequence.
Key Differences Between Eukaryotic and Prokaryotic Transcription
Eukaryotic and prokaryotic transcription share the same basic process of synthesizing RNA from DNA, but they differ significantly in their complexity and regulation. Prokaryotic cells, such as bacteria, have a simpler transcription mechanism due to their less compartmentalized structure. Eukaryotic cells, on the other hand, have a more intricate system due to their larger genomes and the presence of a nucleus. These differences stem from their distinct cellular environments and evolutionary needs.
The transcription process involves several stages: initiation, elongation, and termination. In prokaryotes, this process occurs within the cytoplasm and involves fewer proteins, while in eukaryotes, transcription takes place in the nucleus and requires more regulatory proteins, such as transcription factors. This increased complexity in eukaryotes allows for more precise control of gene expression.
Differences in Transcriptional Mechanisms
- Location:
- Prokaryotes: Transcription happens directly in the cytoplasm.
- Eukaryotes: Transcription occurs in the nucleus.
- RNA Polymerase:
- Prokaryotes: A single RNA polymerase is used for all types of transcription.
- Eukaryotes: Multiple RNA polymerases (I, II, III) are responsible for different types of RNA synthesis (e.g., mRNA, rRNA, tRNA).
- Promoter Elements:
- Prokaryotes: Promoters consist of a -10 and -35 region recognized by the sigma factor.
- Eukaryotes: Promoters are more complex, including the TATA box, enhancers, and other regulatory elements.
Additional Key Differences
- Post-transcriptional Modifications:
- Prokaryotes: mRNA is usually not modified post-transcription.
- Eukaryotes: mRNA undergoes several modifications, including capping, polyadenylation, and splicing.
- Transcription and Translation Coupling:
- Prokaryotes: Transcription and translation can occur simultaneously in the cytoplasm.
- Eukaryotes: Transcription occurs separately in the nucleus, and translation happens in the cytoplasm, so they are not coupled.
Important Note: The ability to regulate transcription more precisely in eukaryotes allows for a greater range of cellular functions and adaptations. This complexity is crucial for multicellular organisms, where different cell types need to express specific genes at different times.
Comparison Table
Feature | Prokaryotic Transcription | Eukaryotic Transcription |
---|---|---|
Location | Cytoplasm | Nucleus |
RNA Polymerase | Single RNA polymerase | Multiple RNA polymerases |
Post-transcriptional Modifications | None | Extensive modifications (capping, splicing, polyadenylation) |
Transcription/Translation Coupling | Yes, simultaneous | No, separate processes |
The Effect of Genetic Mutations on Gene Expression Regulation
Genetic mutations can significantly alter the regulation of gene expression by modifying the DNA sequence or the factors that control transcription. These mutations may result in changes to the promoter regions, enhancers, or silencers, leading to abnormal activation or silencing of genes. Mutations can also affect the stability and function of transcription factors, which are key proteins involved in regulating gene expression. Depending on the location and nature of the mutation, gene expression can either be upregulated or downregulated, potentially leading to diseases or disorders.
One of the most important consequences of such mutations is their potential to disrupt normal cellular processes. Altered expression of certain genes can cause unregulated cell division, impaired response to environmental signals, or defective protein production. Understanding how mutations affect gene regulation is critical for developing treatments for genetic diseases and improving our overall knowledge of molecular biology.
Types of Mutations and Their Effects
- Point Mutations: A single nucleotide change can affect the binding of transcription factors, leading to either enhanced or reduced transcription.
- Insertions or Deletions: These can shift the reading frame or create new splice sites, disrupting normal gene expression.
- Copy Number Variations: Large-scale duplications or deletions of gene regions can lead to an imbalance in gene expression levels.
Mechanisms of Mutation Impact
- Altered Promoter Function: Mutations in the promoter region can either increase or decrease the binding of RNA polymerase, directly impacting gene transcription.
- Disruption of Transcription Factor Binding: Changes in the DNA sequence can prevent transcription factors from binding to their respective sites, reducing the gene’s expression.
- Changes in mRNA Processing: Mutations may cause improper splicing or instability of mRNA, leading to defective protein synthesis.
Impact on Disease Development
Mutations that affect gene regulation can be the root cause of various genetic disorders, such as cancer, developmental disorders, and metabolic diseases. For instance, mutations in tumor suppressor genes or oncogenes can lead to uncontrolled cell growth.
Summary Table of Mutation Effects
Type of Mutation | Effect on Gene Expression | Possible Consequence |
---|---|---|
Point Mutation | Changes in transcription factor binding, possible upregulation or downregulation | Cancer, developmental defects |
Insertion/Deletion | Frame shifts, abnormal splicing | Nonfunctional protein, metabolic disorders |
Copy Number Variation | Imbalanced gene expression | Neurodevelopmental disorders, cancers |
Techniques for Analyzing Transcription and Gene Expression Data
Understanding gene expression is essential for studying various biological processes, such as cellular responses to environmental stimuli, disease mechanisms, and developmental pathways. A variety of techniques are available for analyzing transcription data, each offering distinct advantages in terms of sensitivity, resolution, and throughput. These methods provide insights into the complexity of gene regulation and allow researchers to quantify and compare gene expression levels across different conditions and experimental setups.
Among the most commonly used techniques are high-throughput sequencing, quantitative PCR, and microarrays. Each of these methods plays a critical role in identifying gene activity patterns, enabling the discovery of biomarkers and therapeutic targets. Additionally, the integration of computational tools has significantly enhanced the analysis of gene expression data by facilitating data normalization, visualization, and statistical comparison.
Common Methods for Gene Expression Analysis
- RNA-Seq: A high-throughput sequencing method that provides comprehensive and accurate transcriptome analysis. It measures both the quantity and sequence of RNA molecules in a sample.
- qPCR (Quantitative PCR): A technique that amplifies RNA transcripts into complementary DNA (cDNA) and quantifies gene expression based on fluorescence signals. It is highly sensitive and useful for validating results obtained from RNA-Seq.
- Microarrays: A method that uses a grid of DNA probes to measure the expression levels of thousands of genes simultaneously. It is cost-effective but less sensitive than RNA-Seq.
Data Analysis and Interpretation
- Normalization: Essential for correcting systematic biases in the data (e.g., differences in RNA input, sequencing depth). Techniques like RPKM (Reads Per Kilobase Million) or TPM (Transcripts Per Million) are commonly used.
- Differential Expression Analysis: Identifies genes that show significant differences in expression across different experimental conditions. Popular tools include DESeq2 and edgeR.
- Pathway and Gene Ontology Analysis: Provides biological context by linking differentially expressed genes to known pathways and functional categories. Tools like DAVID and GSEA are frequently used.
Key Considerations in Gene Expression Studies
Method | Advantages | Limitations |
---|---|---|
RNA-Seq | High sensitivity, deep sequencing, detection of novel transcripts | Expensive, complex data analysis |
qPCR | Highly sensitive, fast, and cost-effective for targeted gene analysis | Limited to a small number of genes, less comprehensive |
Microarrays | High-throughput, allows for analysis of thousands of genes | Less sensitive, limited by the probes used |
"The choice of method depends on the research question, the available resources, and the desired resolution of the data. Combining multiple techniques can provide a more robust understanding of gene expression dynamics."
Real-World Applications of Transcription Research in Medicine
Research into the mechanisms of transcription has provided a wealth of insights that are transforming modern medicine. Understanding how genes are expressed at the transcriptional level is crucial for developing targeted therapies and diagnostic tools. One of the key applications is in the treatment of genetic disorders, where manipulating transcription factors can help correct or alleviate the symptoms of diseases caused by faulty gene expression.
In addition, transcription research plays a significant role in the field of cancer therapy. Abnormal gene expression is a hallmark of many cancers, and scientists are developing strategies to target these transcriptional changes. By restoring normal transcriptional regulation or inhibiting the expression of oncogenes, novel treatments are being designed to specifically target cancer cells without affecting healthy tissues.
Applications in Genetic Disorders
One of the most promising areas of transcription research is the treatment of genetic diseases. For example, some therapies aim to correct the misregulation of gene expression in diseases like cystic fibrosis, Duchenne muscular dystrophy, and sickle cell anemia. By manipulating transcription factors, researchers can potentially restore normal gene function or activate compensatory pathways to bypass defective genes.
- Cystic fibrosis: Strategies focus on enhancing the transcription of the CFTR gene to restore proper ion transport in affected cells.
- Duchenne muscular dystrophy: Gene therapies are being developed to promote the transcription of dystrophin in muscle cells, improving muscle function.
- Sickle cell anemia: Researchers are working on ways to activate fetal hemoglobin gene transcription to compensate for defective adult hemoglobin production.
Cancer Treatment and Transcriptional Regulation
In oncology, the regulation of gene expression is a key factor in tumor growth and metastasis. Cancer cells often exhibit dysregulated transcriptional programs that promote uncontrolled cell division. Targeting these abnormal transcriptional networks has become an emerging approach in cancer therapy.
- Inhibition of oncogenes: Researchers are developing small molecules or biologics that specifically target transcription factors that activate oncogenes.
- Restoration of tumor suppressor gene expression: Techniques like gene editing and transcription factor modulation aim to reactivate silenced tumor suppressors in cancer cells.
"Transcriptional regulation is central to understanding the molecular basis of cancer, and the ability to manipulate gene expression holds promise for developing more effective, targeted therapies."
Gene Expression and Personalized Medicine
The growing field of personalized medicine is heavily reliant on the understanding of individual gene expression patterns. Transcription profiling helps clinicians identify specific biomarkers for diseases, allowing for more precise and tailored treatments. By analyzing the transcriptional activity of genes in individual patients, healthcare providers can predict disease progression and select the most effective therapies.
Application | Benefit |
---|---|
Gene expression profiling | Enables personalized treatment plans based on specific genetic activity in patients. |
Transcriptome analysis | Identifies molecular signatures of diseases, aiding in early diagnosis and treatment optimization. |